Contents
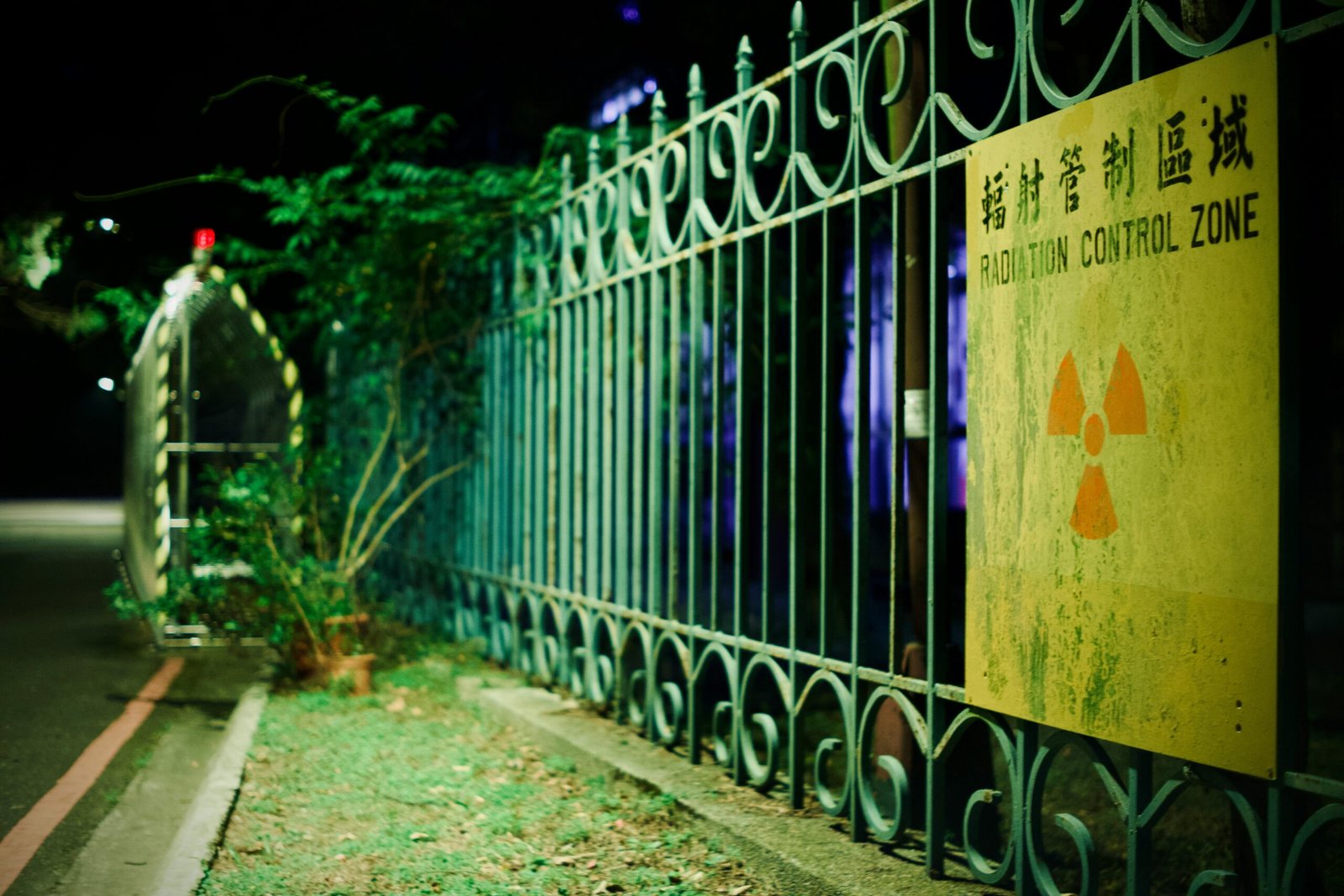
Introduction to Proton Halos
Proton halos represent a fascinating phenomenon in the realm of nuclear physics, where a nucleus exhibits a diffuse cloud of protons extending beyond its traditional boundary. These structures are characterized by one or more protons that are weakly bound to the nucleus, forming a ‘halo’ around a denser core. This unique configuration challenges conventional understanding of nuclear forces and the stability of atomic nuclei.
Proton halos are significant for several reasons. Firstly, their existence provides critical insights into the behavior of protons under extreme conditions, which is essential for refining theoretical models of nuclear structure. Traditional models often assume a more compact and uniformly distributed nuclear matter, but the discovery of proton halos necessitates adjustments to account for these extended distributions. This, in turn, influences our comprehension of nuclear interactions and the forces at play within an atomic nucleus.
Furthermore, understanding proton halos is crucial for experimental nuclear research. Precision measurement programmes aimed at studying these phenomena have unveiled new aspects of nuclear reactions and decay processes. These programmes utilize sophisticated detectors and advanced techniques to observe and measure the properties of proton halos with high accuracy. The data obtained from such experiments not only validate theoretical predictions but also drive the development of new theories to explain the observed anomalies.
In addition, proton halos have implications for astrophysics, particularly in the context of nucleosynthesis—the process by which elements are formed in stars. The presence of proton halos can affect the reaction rates and pathways in stellar environments, thereby influencing the abundance of elements in the universe. This connection underscores the broader significance of studying proton halos, as it links microscopic nuclear phenomena with macroscopic cosmic events.
Overall, the investigation of proton halos represents a vital frontier in nuclear physics, bridging gaps between theoretical predictions and experimental observations, and enhancing our understanding of the fundamental forces that govern atomic nuclei.
Proton halos, a captivating phenomenon in nuclear physics, have intrigued scientists for decades. The quest to understand these diffuse nuclear structures has its roots in the early 20th century, following the discovery of the atomic nucleus by Ernest Rutherford. Initial attempts to study the nucleus primarily focused on its overall composition and properties, but as experimental techniques advanced, researchers began to uncover more nuanced aspects of nuclear structure, including the presence of proton halos.
One of the pivotal moments in the study of proton halos came in the 1980s, with the advent of radioactive ion beam facilities. These facilities enabled the creation and investigation of exotic nuclei far from stability, which often exhibit halo characteristics. The groundbreaking discovery of neutron halos in light, neutron-rich nuclei spurred interest in the possibility of proton halos. This was a period marked by significant theoretical and experimental efforts to explore and understand these elusive structures.
Early experiments faced numerous challenges due to the fleeting and delicate nature of halo nuclei. Proton halos, in particular, were difficult to study because protons experience a repulsive Coulomb force, making it less straightforward to form a diffuse halo structure compared to neutrons. Advanced detection techniques and refined theoretical models were essential in overcoming these obstacles. Key discoveries, such as the identification of proton halos in isotopes like 8B and 17F, underscored the complexity of nuclear forces and the novel behaviors exhibited by these exotic nuclei.
Throughout the years, various theoretical models have been proposed to explain the formation and stability of proton halos. These models have evolved in tandem with experimental evidence, highlighting the interplay between theory and experiment in nuclear physics. The continuous development of precision measurement programmes has further enriched our understanding, allowing for more accurate and detailed studies of proton halos. This historical journey, marked by innovation and collaboration, has paved the way for ongoing research and the future exploration of nuclear structures.
The Role of Precision Measurement Programmes
In recent years, precision measurement programmes have significantly advanced our understanding of proton halos. These programmes leverage cutting-edge technology and innovative methodologies to achieve unprecedented accuracy in data collection and analysis. By utilizing state-of-the-art detectors, advanced computational models, and high-resolution imaging techniques, researchers can now observe and measure the subtle properties and behaviors of proton halos with remarkable precision.
One major technological advancement that has propelled these programmes forward is the development of next-generation particle accelerators. These accelerators generate high-energy collisions that produce proton halos, allowing scientists to study their properties in greater detail. Additionally, the use of sophisticated detectors and sensors has enhanced our ability to capture minute variations in proton behavior, enabling more accurate measurements of their spatial distribution and energy levels.
Another critical component of these precision measurement programmes is the implementation of advanced computational techniques. High-performance computing platforms and machine learning algorithms are employed to analyze vast datasets, identifying patterns and correlations that would be impossible to discern manually. These computational tools not only improve the precision of measurements but also accelerate the pace of research by automating complex data analysis processes.
Furthermore, high-resolution imaging techniques, such as electron microscopy and tomography, have been instrumental in visualizing proton halos at an atomic level. These imaging methods provide detailed insights into the structural and dynamic characteristics of proton halos, revealing information about their formation, stability, and interactions with other particles. By combining these imaging techniques with precision measurement data, researchers can develop more accurate models of proton behavior and refine our theoretical understanding of nuclear physics.
Overall, the integration of advanced technologies and methodologies in precision measurement programmes has revolutionized the study of proton halos. These programmes continue to push the boundaries of what is possible in nuclear physics, providing new insights into the fundamental nature of matter and paving the way for future discoveries.
Key Findings from Recent Research
Recent advancements in precision measurement programmes have shed new light on the enigmatic nature of proton halos, significantly enhancing our understanding of their structure and behavior. These high-precision experiments have uncovered intricate details about the distribution of protons within these halos, revealing complexities that were previously unobservable.
One of the most notable findings emerged from experiments conducted at the RIKEN Center for Accelerator-Based Science in Japan. Utilizing advanced detection techniques, researchers were able to measure the spatial distribution of protons in halo nuclei with unprecedented accuracy. These measurements demonstrated that proton halos exhibit a diffuse cloud-like structure, where protons are loosely bound and extend far beyond the nuclear core. This discovery challenges traditional nuclear models, which assumed a more compact distribution of nucleons.
Additionally, research at the European Organization for Nuclear Research (CERN) has contributed significantly to our understanding of proton halos. By employing the ISOLDE (Isotope Separator On-Line Device) facility, scientists were able to produce exotic halo nuclei and measure their properties with high precision. These experiments revealed that proton halos are highly sensitive to the underlying nuclear forces, which has profound implications for our theoretical models of nuclear interactions.
Another groundbreaking study conducted at the Facility for Rare Isotope Beams (FRIB) in the United States focused on the behavior of proton halos under extreme conditions. By accelerating halo nuclei to high energies and observing their interactions, researchers discovered that the halos can exhibit unexpected stability, even in highly dynamic environments. This finding has potential applications in understanding astrophysical processes, such as nucleosynthesis in supernovae, where proton-rich environments play a crucial role.
Overall, these precision measurement programmes have provided invaluable data that not only refine our current nuclear models but also open new avenues for research. The insights gained from these studies are poised to revolutionize our comprehension of nuclear matter and the fundamental forces that govern its behavior.
Implications for Nuclear Physics
The recent advancements in the precision measurement programmes of proton halos have far-reaching implications for the broader field of nuclear physics. A more nuanced understanding of proton halos can significantly influence various areas of research, including nuclear reactions, astrophysics, and the development of nuclear models.
In the realm of nuclear reactions, the study of proton halos offers valuable insights into the behavior of nuclei under extreme conditions. Proton halos, characterized by their diffuse and extended nature, challenge existing nuclear models and provide a unique opportunity to test theoretical predictions. By examining how these halos interact with other nuclei, researchers can refine their understanding of nuclear forces and reaction mechanisms, leading to more accurate predictions of reaction rates and cross-sections. This has practical implications for applications such as nuclear energy production and the synthesis of new elements.
Astrophysics also stands to benefit from the enhanced understanding of proton halos. The processes that govern stellar evolution, supernova explosions, and nucleosynthesis are deeply intertwined with nuclear reactions involving exotic nuclei. Proton halos, with their unusual structure, can influence the rates of key reactions that occur in these astrophysical environments. By incorporating data from precision measurements of proton halos into astrophysical models, scientists can improve their predictions of stellar behavior and the chemical evolution of the universe.
Furthermore, the development of nuclear models is poised for significant advancements. Traditional nuclear models often rely on simplifying assumptions that may not fully capture the complexities of halo nuclei. The detailed data obtained from precision measurement programmes allows for the refinement of these models, leading to a more accurate representation of nuclear structure and dynamics. This, in turn, enhances our ability to predict the properties of unknown or yet-to-be-discovered isotopes, guiding future experimental investigations.
Overall, the study of proton halos represents a crucial frontier in nuclear physics. It not only deepens our understanding of nuclear matter but also has the potential to transform our approach to a wide range of scientific and practical challenges. The insights gained from this research will undoubtedly continue to shape the landscape of nuclear physics for years to come.
Technological Innovations and Instrumentation
Technological advancements in the field of nuclear physics have significantly enhanced our understanding of proton halos. The advent of precision measurement programmes has been facilitated by cutting-edge instrumentation and groundbreaking innovations. These new tools have allowed scientists to explore nuclear structures with unprecedented accuracy and detail, providing insights that were previously unattainable.
One such advancement is the development of high-resolution gamma-ray detectors. These detectors are capable of identifying and measuring gamma rays emitted during nuclear reactions with exceptional precision. By capturing these emissions, researchers can deduce the energy levels and configurations of protons within the nucleus. This technology represents a substantial leap over older, less sensitive detectors that were unable to capture the nuanced data required for studying proton halos.
Another significant innovation is the use of ion traps and storage rings. Ion traps allow for the confinement and manipulation of charged particles, including protons, under controlled conditions. Storage rings, on the other hand, enable the circulation of these particles at high velocities, facilitating extended observation periods. These instruments have been instrumental in isolating specific particles and studying their behavior in a way that traditional particle accelerators could not achieve.
Additionally, the implementation of advanced computational methods has revolutionized data analysis in proton halo research. High-performance computing systems and sophisticated algorithms can process vast amounts of experimental data, modeling nuclear interactions with high fidelity. This computational power allows for the simulation of complex nuclear phenomena, offering theoretical predictions that guide experimental investigations.
These technological innovations collectively mark a significant advancement over previous methodologies. By providing higher precision, greater control, and more detailed data, they have opened new avenues for exploring the enigmatic nature of proton halos. These tools not only enhance our understanding of nuclear physics but also pave the way for future discoveries in the realm of subatomic particles.
Future Directions in Proton Halo Research
Advancing the understanding of proton halos remains a pivotal endeavor in modern nuclear physics. Future research directions are poised to leverage both cutting-edge technology and innovative experimental designs to unravel the mysteries of these quantum phenomena. Upcoming experiments are set to push the boundaries of our knowledge, employing high-precision measurement techniques and novel detection systems.
One of the most anticipated experiments involves the use of radioactive ion beam facilities, such as the Facility for Rare Isotope Beams (FRIB) and the Radioactive Isotope Beam Factory (RIBF). These facilities are designed to produce and study rare isotopes, including those that exhibit proton halo characteristics. By generating isotopes at energies and intensities previously unattainable, researchers aim to capture detailed data on halo nuclei, thus providing deeper insights into their structure and behavior.
Technological advancements in detector systems are also on the horizon. Innovations such as advanced gamma-ray detectors and next-generation silicon detectors are expected to enhance the accuracy and resolution of measurements, allowing for more precise characterizations of proton halos. These tools will facilitate the identification of subtle interactions within halo nuclei, shedding light on the forces and mechanisms at play.
Moreover, the development of sophisticated theoretical models will be crucial in complementing experimental efforts. Enhanced computational capabilities and refined nuclear theories aim to predict the properties of proton halos with greater accuracy. Researchers are particularly focused on understanding the role of the nuclear force and the impact of proton-neutron correlations within these exotic nuclei.
Key questions driving future research include the exact nature of the forces that govern proton halos, the conditions under which they form, and their implications for our broader understanding of nuclear matter. By addressing these questions, scientists hope to bridge gaps in our comprehension of the fundamental principles that underpin the atomic nucleus.
The road ahead in proton halo research promises to be both challenging and enlightening. As experimental techniques and theoretical frameworks converge, the scientific community stands on the cusp of significant breakthroughs, poised to unlock the secrets of these fascinating quantum structures.
Conclusion
In this blog post, we have delved into the intricate world of proton halos, unraveling the complexities that define these elusive nuclear phenomena. Precision measurement programmes have played a pivotal role in advancing our understanding, enabling scientists to observe and quantify properties of proton halos with unprecedented accuracy. These meticulous measurements have not only substantiated theoretical models but have also provided empirical data essential for refining our knowledge of nuclear forces and interactions.
As we have discussed, the insights gained from precision measurements extend beyond the realm of proton halos. They have the potential to significantly influence the broader field of nuclear physics, offering new perspectives on the structure and behavior of atomic nuclei. The refined data can enhance our comprehension of nuclear reactions, which is crucial for applications ranging from energy production in nuclear reactors to the synthesis of elements in astrophysical environments.
Furthermore, the advancements in precision measurement techniques themselves are noteworthy. The continual improvement of experimental methodologies and technologies ensures that future research will yield even more detailed and accurate findings. This progress is not just a testament to human ingenuity but also a driving force that propels scientific inquiry forward, opening doors to new discoveries and innovations.
Looking ahead, the implications of these findings are vast. As our grasp of proton halos becomes more comprehensive, we can expect ripple effects across related disciplines such as particle physics, quantum mechanics, and materials science. The interplay between experimental data and theoretical frameworks will continue to shape our understanding of the universe at its most fundamental level, highlighting the enduring importance of precision measurement programmes in scientific exploration.
OUR SITE: toinewsalert.com